You may not have heard of them. You may not be able to see them with the naked eye. But diatoms—single-celled algae found in fresh and marine waters—are among the most important organisms we share the planet with.
Like their cousins, the land-based plants, diatoms convert sunlight and carbon dioxide into energy via photosynthesis.
“Every fifth breath we take we owe to diatoms,” explains Thomas Mock, Professor and marine microbiology expert at the University of East Anglia. Like land-based plants, diatoms store carbon in their bodies and play a significant role in “locking carbon” in the deep ocean. If you follow the ecological processes thereafter and far enough, diatoms continue to make their mark on human life in myriad ways.
Structurally speaking, diatom bodies are sort of like land-based plants, but with one key difference. “You can imagine that diatoms are similar to trees. They have a really strong bark, but the bark is not made of cellulose—the bark is made of silica,” Mock says. These silica-based cell walls give rise to some of the most beautiful, intricate forms.
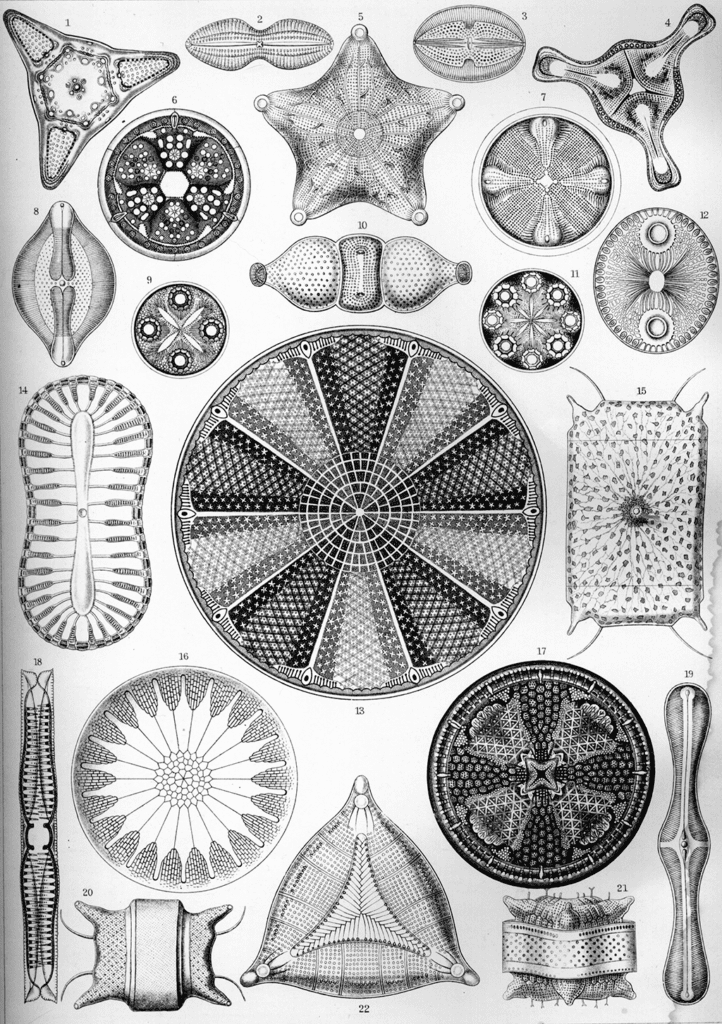
Diatoms, Art Forms in Nature by Ernst Haeckel | Wikimedia Commons
A mesmerisation of natural variation, diatom morphology is appreciated for its artistic qualities. “In Victorian times, people who could afford microscopes took up arranging diatoms by the different patterns,” says Irena Kaczmarska, Professor and diatom researcher at Mount Allison University.
Diatom morphology isn’t just a marvel to enchant the imagination. It lies at the heart of taxonomy, the key for identifying one genus or even species from another.
Cataloguing diatoms, one valve at a time
The crux of identification is the diatom’s valve. “In diatoms, the frustule [cell wall] is very complex, made up of two sections called valves. They fit together like the bottom and top of a petri dish,” Kaczmarska explains.
Conservative estimates place the number of diatom species somewhere between 100,000 and 200,000. Even with improved technology, we have only identified around 20,000 species that fall into one of two broad groups: centric and pennate.
Centric diatoms are radially symmetric, with a pattern that starts from a central point and works its way outwards, like a starfish. Pennate diatoms are bilaterally symmetric, with a pattern that starts down a central line, like a feather. Pennate diatoms are more elongated and less compact than centric diatoms. Most of the time, diatoms are relatively easy to categorise as centric or pennate. But there are exceptions.
Ardissonea crystallina has a decidedly pennate morphology, but molecular examination suggests that it may be a centric, albeit one that is more elongated than usual. A. crystallina’s classification is currently the subject of hot debate, one which Kaczmarska and an international team of collaborators recently weighed in on.
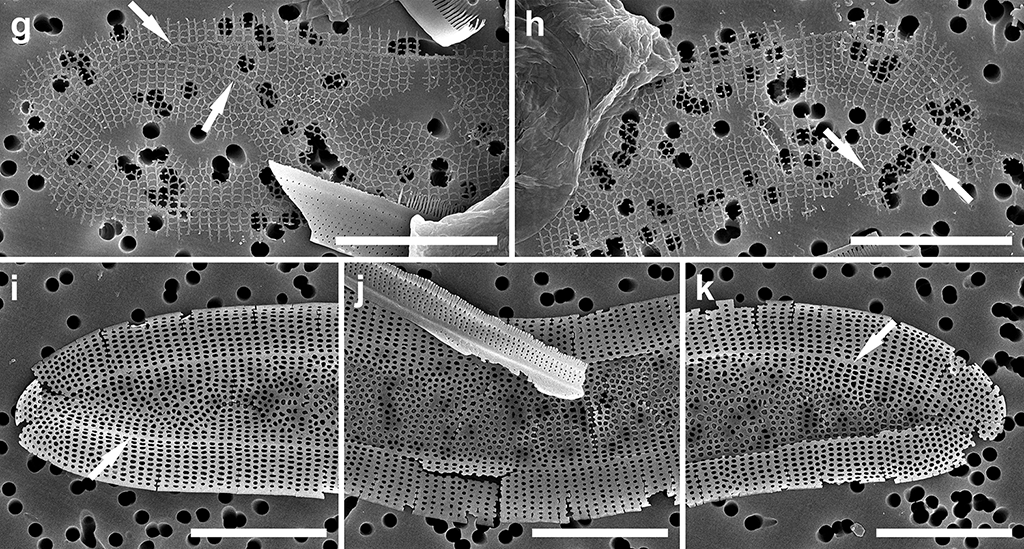
Ardissonea crystallina, variations in development of the valve | Kaczmarska et al. 2020
The key, Kaczmarska says, is in studying the entire developmental process of the diatom’s valve. By comparing the earliest stages of A. crystallina’s valve development against two indisputably centric diatoms, Kaczmarska found that the molecular studies are most likely the correct ones—A. crystallina is a centric diatom.
Kaczmarska’s findings—published in the journal Botany—are not only relevant for diatom taxonomy, but also for making evolutionary inferences in general; “We should look at the entire development process from the earliest stages to the latest, and not rely on the finished product,” she says.
Shaping up environmental assessments
As one might imagine, identifying diatom species is an extraordinarily meticulous process, something that Joseph Bennett, Professor at Carleton University, has first-hand experience of.
“When I was an undergraduate, I did my honours thesis on diatoms, salinity, and climate change. Then I did a master’s degree on a similar topic. I spent hundreds of hours looking down a microscope at these gorgeous creatures. I love their intricate, beautiful shapes, but they’re hard as hell to identify.”
Bennett spent a great deal of time getting help from other people, pouring into books, and even trying to read taxonomic identification advice in German. “I had to use a German–English dictionary and a rough translation guide produced by the lab for technical terms. It was not fun.”
Later Bennett worked as a consultant where he specialised in identifying diatoms for environmental assessments. “Diatom species have a very strong fidelity to certain environments, so looking at diatom assemblages is really useful for assessments,” Bennett says. “I was thinking about how we can get the best signal out of diatoms, without the need for specialised taxonomists, who are pretty rare creatures.”
Research lead by one of Bennett’s honours students Emily Cormier and published in the Canadian Journal of Fisheries and Aquatic Sciences suggests that the solution may lie in the simplicity of shapes.
Beyond the centric–pennate grouping, diatom species come in all sorts of shapes. Some of these shapes seem to be related to adaptations to environmental conditions. “There are certain types of diatoms that are shaped bendy, like a banana, that as a group are really good at handling acidic environments,” Bennet explains.
The scientists put together a “morphological trait” guide designed for non-specialists. Then, using existing data sets, they ran a series of assessments using both the trait and the traditional species assemblage approach.
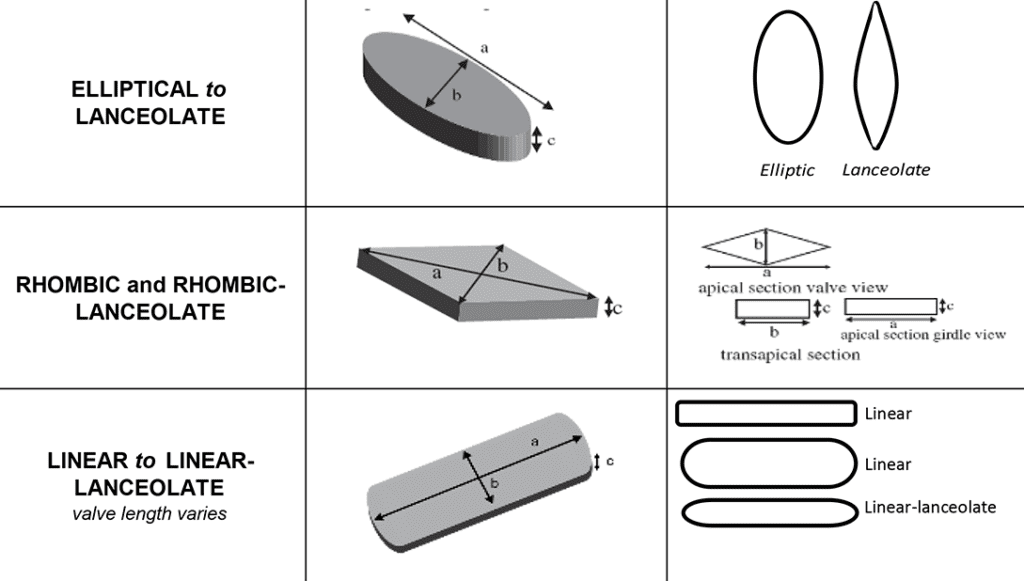
Excerpt from the morphological trait guide | Cormier et al. 2020
When the results came in, Bennett was pleasantly surprised. Although the traditional species assemblage approach did perform better, the shape-based approach did not lag far behind. “I thought [that] using very coarse shapes was not going to lead to the level of predictive ability we got,” Bennett recalls. “Instead of throwing out the diatoms because you have no access to a specialist, you can look at their shapes, and provide a line of evidence that can support conclusions.”
Following the trail of digested diatoms
Being a type of phytoplankton, diatoms underpin some of the most productive and diverse aquatic food webs. Phytoplankton sit at the bottom of the food web, consumed by one small species, which is then eaten by another, then another, then another. Crucially, phytoplankton—including diatoms—produce fatty acids, like omega-3.
“Most organisms like fish cannot synthesise fatty acids–they have to acquire it through diet,” explains Camilla Parzanini, a postdoctoral researcher at Ryerson University.
These fatty acids aren’t necessarily lost in the first animal to eat the diatoms but are often stored in that animal’s body. When that animal is itself eaten, the fatty acids move into its predator’s body. “Sometimes the fatty acids undergo some transformation as they move through one animal to another, but it’s something that you can detect,” adds Parzanini.
Different phytoplankton species have different ratios of fatty acids, and different environments have different groups of species. In her recently published paper in Environmental Reviews, Parzanini shows that, broadly speaking, marine and freshwater fish have very different fatty acid profiles. These profiles can also change by latitude in response to varying conditions like sea temperature; this, Parzanini explains, means fatty acids are useful indicators of where an animal has been.
Knowing where a fish has been eating has many benefits, such as managing migratory species or in the fight against seafood fraud.
“Sometimes we are sold fish that we are told is one species when in reality it is something completely different,” Parzanini points out. “Even marine fish are sold as freshwater fish and vice versa.” Fatty acid profiles can be used alongside DNA evidence to uncover seafood fraud, but they can also act as useful indicators by themselves.
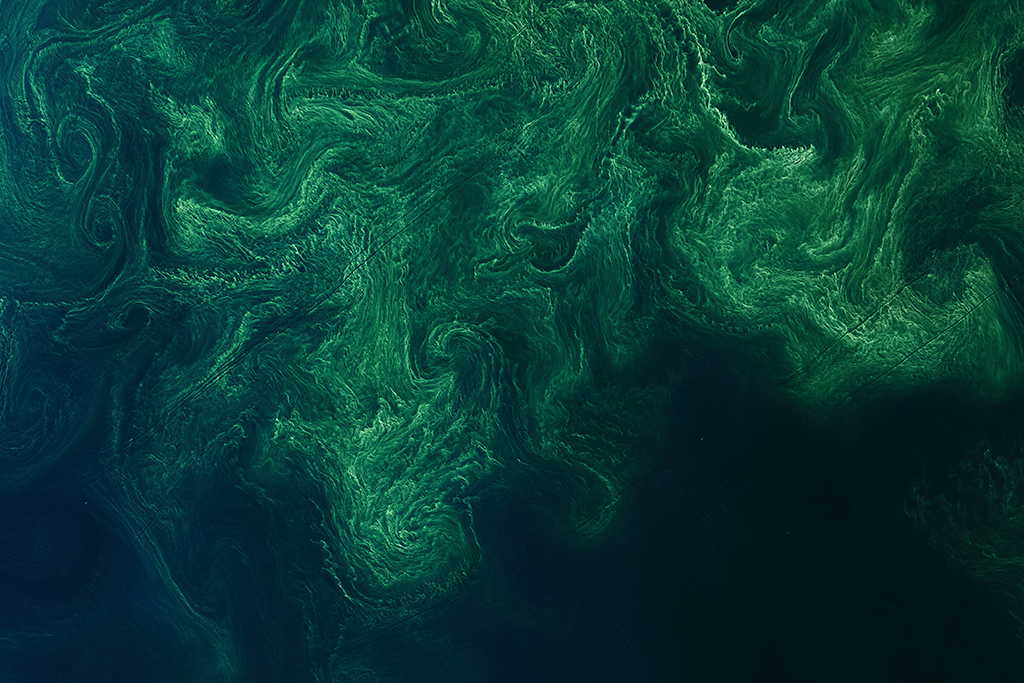
Phytoplankton bloom in the Baltic Sea | NASA’s Earth Observatory
Detailing the diatom domain
Parzanini is currently collating a database of fatty acid profiles from aquatic species ranging from primary producers like diatoms all the way up to the top predators.
“We would like to be able to connect all the different components of the food web,” Parzanini says. “It’s a fine balance between making large-scale inferences and applying that to specific species. You have to be mindful to look into deeper detail.”
Mock is also focusing on uncovering details. With an international team of collaborators, Mock has started the “100 Diatom Genomes Project,” which, as the name suggests, aims to decode the genomes of 100 diatom species. Eventually these genomes could help unravel some of the diatom’s secrets. “What caused the radiation of so many species in just one group?” asks Mock. “We hope to get an idea of how genomic diversification took place over about 200 million years.”
Alongside aiding in evolutionary research and taxonomic grouping, like that conducted by Kaczmarska, the 100 Diatom Genomes project may also support trait-based approaches, such as Parzanini’s work with fatty acid profiles or Bennett’s work with morphology.
As Mock ponders: “We know very little about the genes involved in making shells [cell walls]. We barely know anything about how you create these nanopatterns based on the gene set that is available. Maybe it’s not even related to any proteins. Maybe it’s related to something else. We don’t know.”